Measurement and Control of UFPs of Increasing Importance
Ultrafine particle (UFP) monitoring, as typified by cleanliness control in clean rooms, has become indispensable in a broad range of industries. A typical measurement method is based on laser light scattering. As shown in the figure below, ultrafine particles (UFP) to be measured are transported by a gas or liquid phase flow, and the laser beam is irradiated across the flow. The scattered light generated when particles are present is measured with a photo-detector.
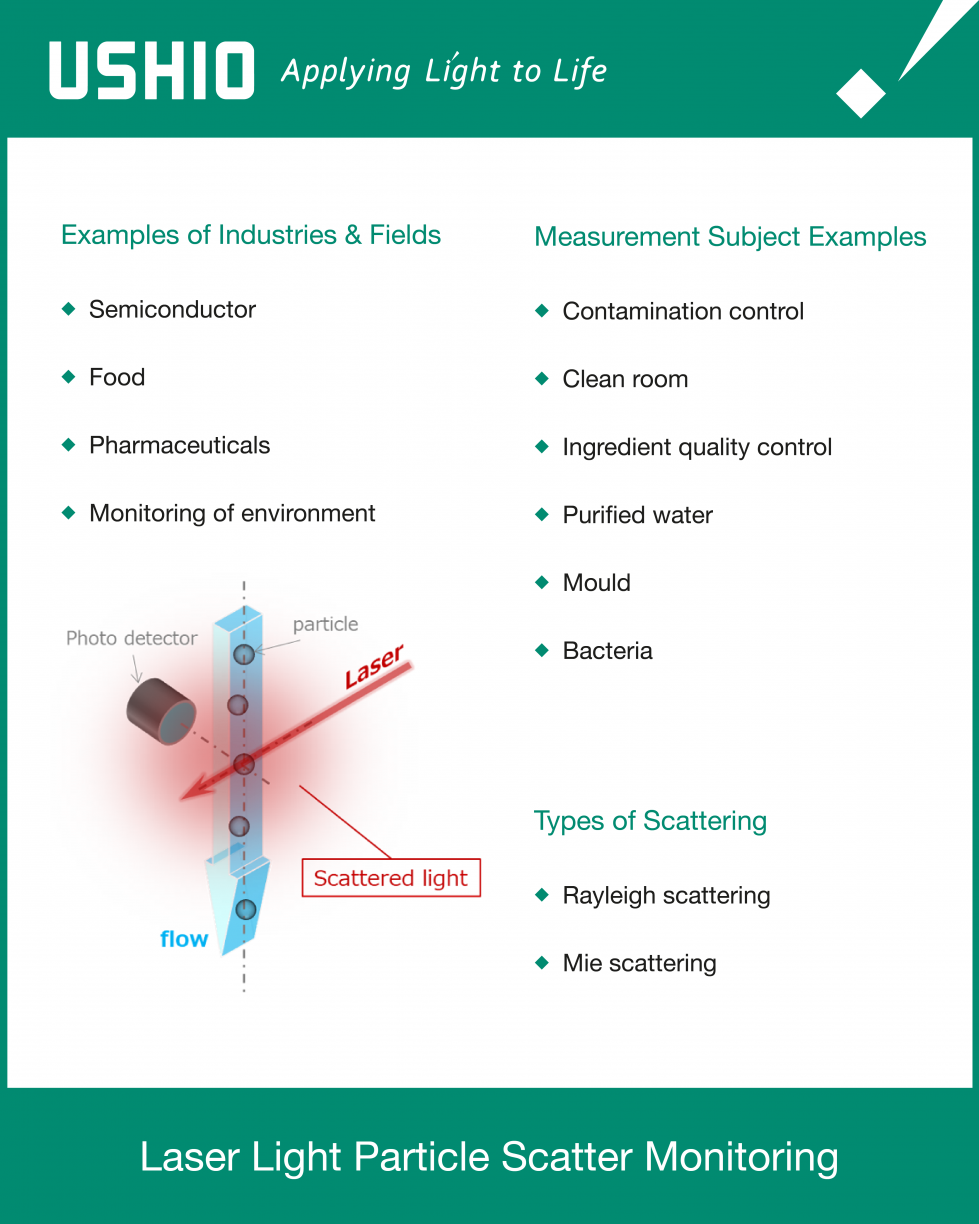
In the semiconductor industry, the size of contamination that can cause a problem is becoming extremely small due to the progress of miniaturization, and monitoring of ultrafine particles measuring only several tens of nanometres in size is becoming necessary. In the pharmaceutical and food industries, particles are counted and controlled as a means of preventing bacterial and other microbiological contamination, but stricter control is required in response to growing consumer awareness.
Scattering in the Rayleigh scattering region and required laser illumination
It is known that light scattering by particles differs greatly depending on the size of the particles relative to the wavelength. This phenomenon is well-defined by the Rayleigh scattering theory: when the UFPs are sufficiently smaller than the wavelength; or by the Mie scattering theory, when the particle and light wavelength are of a similar size.
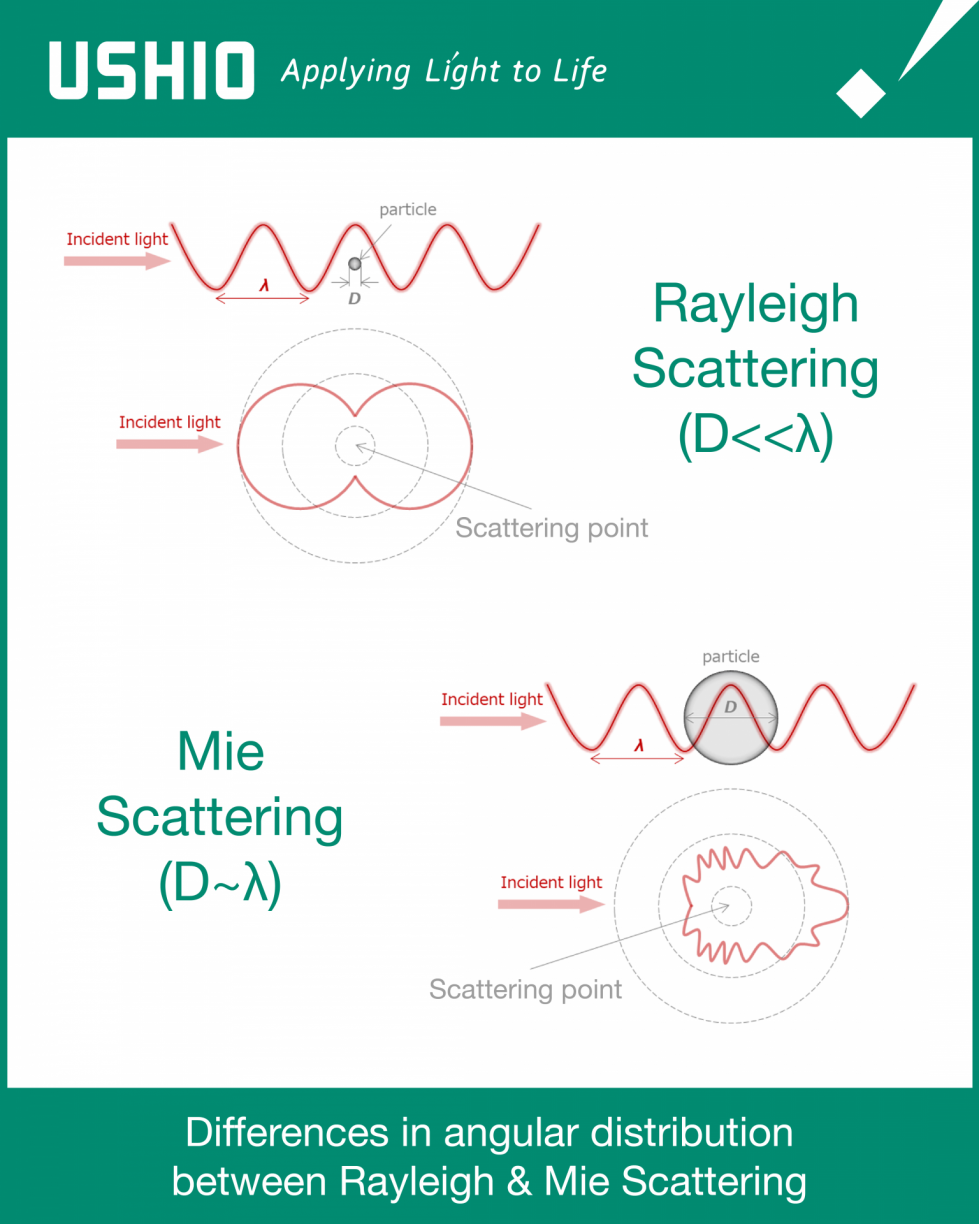
In the Mie scattering region, forward scattering (scattering in the direction of the incident light) is dominant, but the angular distribution of scattered light becomes a complex pattern, which mirrors the shape of the particle that causes the scattering due to interference between scattered light. In the Rayleigh scattering caused by UFP, on the other hand, forward and backward scattering predominate; however, this is dependent on the polarization of the laser light, so care must be taken with the direction of polarization of the laser light (see below). In general, the smallest particle size to be measured is much smaller than the wavelength of the laser light, and is measured in the Rayleigh scattering region.
The Rayleigh scattering intensity (I) is expressed by the following equation:


I1 , I2 are the scattering intensities with polarizations perpendicular and parallel to the scattering plane (the plane defined by both incident and scattered light vectors), respectively; I1o , I2o are the incident light intensities for the respective polarizations; r, n are the particle radius and refractive index; λ is the incident light wavelength; d is the distance from the scattering point to the observation point; θ is the scattering angle (angle formed by incident and scattered light vectors)
From this equation, we first see that the scattering intensity is proportional to the 6th power of the grain size (r), i.e., the scattering intensity rapidly decreases as the grain size decreases (the scattering intensity decreases to 1/64 when the grain size is halved).
Similarly, the scattering intensity is inversely proportional to the 4th power of the incident light wavelength (λ), indicating that shortening the incident light wavelength is effective in increasing the scattering intensity. For example, if the wavelength is 850 nm, the scattering intensity can be increased by a factor of 2.75 if the wavelength is 660 nm, and by a factor of 19.4 if the wavelength is 405 nm. In addition, since the theoretical limit of the laser’s focused spot size is proportional to the wavelength, short-wavelength light is also advantageous in terms of increasing the light flux density to compensate for weak scattering; however, changing the wavelength requires consideration of the characteristics of the photo-detector and the effects of fluorescence generation.
With respect to polarization, the scattering intensity when the polarization direction is perpendicular to the scattering plane (I1 ) has no scattering angle (θ) component, i.e. it scatters isotropically, whereas when the polarization is horizontal to the scattering plane (I2 ) is proportional to cos2 θ, so for example the side scattering (θ = 90°) component is zero. If the photo-detector is placed in the side scattering detection position, only incident light with perpendicular polarization will contribute to the measurement. Ushio’s laser diodes have polarization direction information described as TE mode/TM mode (polarization direction horizontal/perpendicular to the active layer of the laser diode, respectively) in the data sheet for each product.
Examples of laser products suitable for light scattering particle monitoring
Among Ushio’s many choices of laser diodes, there are a few examples of products suitable for light scattering particle monitoring. Since the scattering intensity rapidly decreases as the particle size decreases, illumination with the highest possible flux density is required, and a single-mode, high-power laser is suitable.
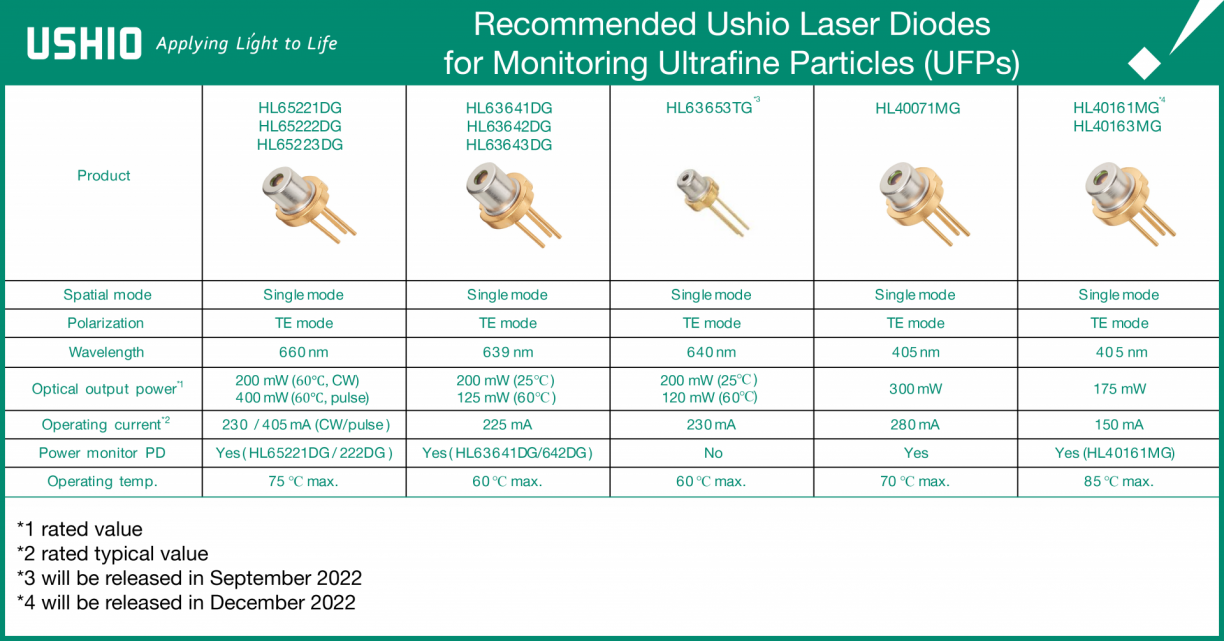
The 660 nm wavelength band has been widely used for DVDs, and many measuring instruments and sensors have been designed with optics in this wavelength band and are still widely used. The HL65221DG series is the latest laser diode developed to achieve high efficiency and high output power in the 660 nm band even in high temperature operation. It boasts an optical output power of 200 mW (CW) and 400 mW in pulsed operation. Operation at temperatures up to 75°C is possible.
The HL63641DG series is a single-mode laser diode with a wavelength of 639 nm and a high power of 200 mW. The latest design improves power efficiency from 28% to 33%, contributing to energy saving and simplifying thermal design of the system. The HL63653TG, also a 640 nm single-mode laser diode, achieves high power of 200 mW in a small package with a diameter of 3.8 mm, contributing to downsizing and space saving of devices.
The HL40071MG and HL40161MG series of 405 nm wavelength laser diodes have the highest optical output power of 300 mW and 175 mW (CW), respectively, for single mode. The HL40161MG series, in particular, is capable of operating at temperatures up to 85°C. These products are suitable for ultrafine particle monitoring, measurement using short-wavelength light, and fluorescence excitation in the biomedical field.
In addition to the products introduced here, Ushio offers a broad lineup of laser diodes to meet your needs. For details, please visit the Ushio Laser Diodes page. Further information about Ushio’s full product range can be found on our Downloads page.